Stem cells represent one of modern medicine’s most promising frontiers, offering unprecedented potential to regenerate damaged tissues and treat degenerative diseases. These undifferentiated cells are characterized by their self-renewal qualities and ability to differentiate into specialized cell types. Several classes of stem cells exist and are grouped both by potency and origin:
- Pluripotent stem cells can differentiate into cell lines from all 3 embryonic germ layers and include embryonic stem cells (ESCs) and induced pluripotent stem cells (iPSCs). Mouse ESCs are derived from the inner cell mass of a preimplantation blastocyst (3.5-day embryo), whereas iPSCs are derived from somatic cells in laboratories through genetic reprogramming using transcription factors such as OCT4, SOX2, KLF4, and c-MYC.1
- Multipotent stem cells, such as mesenchymal stem cells (MSCs) and hematopoietic stem cells (HSCs), may differentiate only within a specific cell lineage.2,3
- Adult stem cells persist throughout the body in various locations including bone marrow, adipose tissue, and the eye, where they maintain and repair cells.
Stem cell therapy is a medical approach that harnesses the differentiation capability of stem cells to treat diseases and repair damaged tissues by replacing the dysfunctional specialized cells with new, healthy cells. In ophthalmology, stem cell therapy has emerged as a transformative strategy for retinal diseases previously considered incurable. By replacing dysfunctional or lost cells, this approach holds promise for conditions like age-related macular degeneration (AMD) and retinitis pigmentosa (RP), in which degeneration of photoreceptors or retinal pigment epithelium (RPE) leads to irreversible vision loss (Figure 1).4
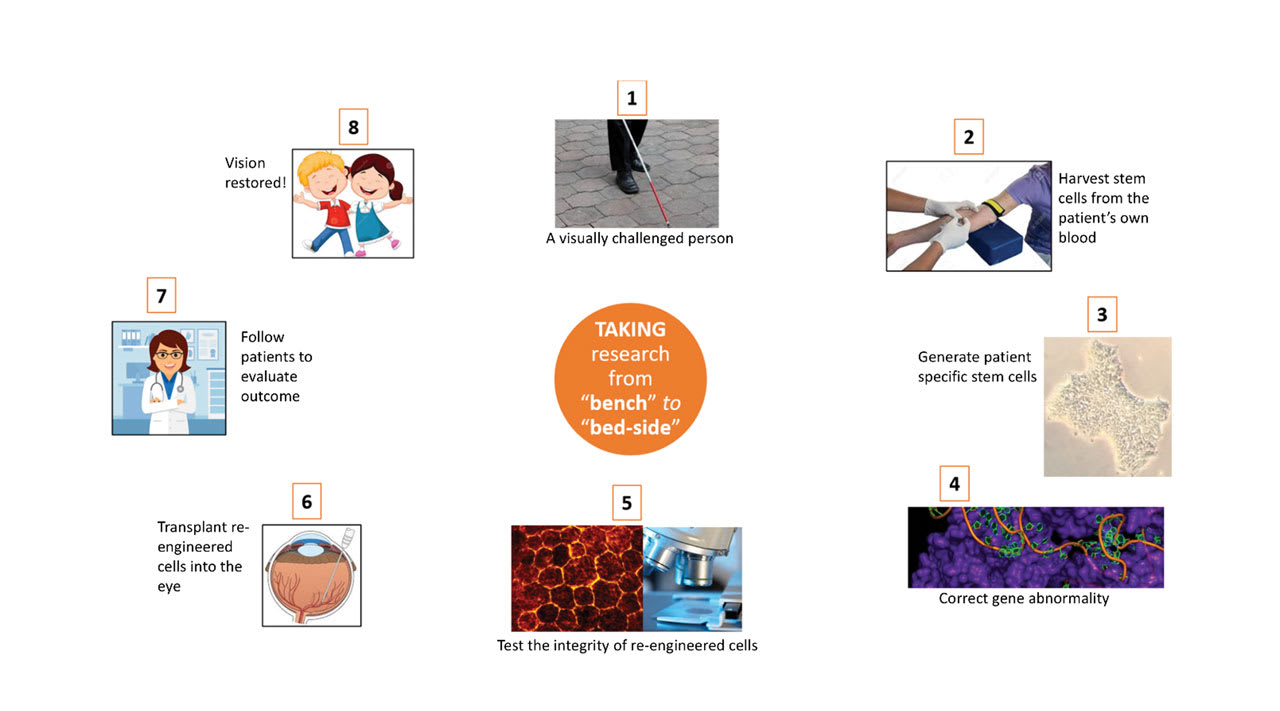
Figure 1. In patient-derived stem cell therapy, induced pluripotent stem cells are harvested and genetically corrected using CRISPR-based genome editing to repair disease-causing mutations. The cells are then differentiated and mature into retinal pigment epithelial (RPE) cells on a biodegradable scaffolding to be retransplanted into the patient’s eye. Image courtesy Ingrid U. Scott, MD, MPH.
The retina has several unique advantages that render it an ideal target for stem cell interventions, including immune privilege, surgical accessibility, and ease of monitoring through advanced imaging. This review provides a broad overview of the current methods and applications of stem cell therapy in retinal diseases, and also discusses limitations and future directions of the practice in ophthalmology.
Stem Cell Applications in Retinal Diseases
The RPE is an essential custodian of photoreceptor health, because it performs phagocytosis of photoreceptor outer segments and regulates metabolism, among other functions.5 Dysfunction or loss of RPE cells is implicated in several retinal diseases, most notably AMD, making RPE replacement a primary target for stem cell therapies.
Given the essential role of RPE cells in photoreceptor maintenance, efforts to replace dysfunctional RPE using pluripotent stem cells have been a focus of clinical research. Humayun et al conducted a phase 1/2a clinical trial using the CPCB-RPE1 implant, which consists of human embryonic stem cell (hESC)–derived RPE cells cultured on a parylene membrane scaffold.6 The authors affirm the safety of the implant and surgical procedure used, as well as the necessity of an immunosuppression regimen to avoid rejection. Although most stem cell therapies focus on dry AMD, Mandai et al reported the successful transplant of autologous iPSC-derived RPE cells in a patient with neovascular (wet) AMD.7 These findings were particularly significant given that approaches for wet AMD must also overcome the surgical challenges associated with the presence of choroidal neovascularization (CNV) and related scarring. The patient reported by Mandai et al received a suspension of iPSC-derived RPE cells via subretinal injection, with no evidence of rejection or tumor formation at 12 months following the operation. Although no significant improvement in visual acuity was observed, the study attested to the utility of the approach even in patients with wet AMD.
In a parallel advancement, the London Project to Cure Blindness reported on a phase 1 study using hESC-derived RPE patch for wet AMD.8 Here, da Cruz et al demonstrated improved visual acuity in patients, adequate integration of the patch with host tissue, and no evidence of rejection or tumor formation at 12 months. Complementing these results, Davis et al found that cells at intermediate stages of differentiation (approximately 4 weeks of culture) demonstrated increased efficacy for functional rescue in rat models of retinal degeneration compared to cells at earlier (2 weeks culture) or later (7-8 weeks culture) differentiation stages.9 Collectively, these studies have helped to establish the feasibility of the transplant approach, but further research will be necessary to improve and quantify the efficacy of this method.
In 2021, the New York Stem Cell Foundation (NYSCF), in collaboration with Columbia University, announced a stem cell therapy initiative targeting dry AMD.10 The treatment uses a protocol developed by Kapil Bharti, PhD, senior investigator at the National Eye Institute, which is similar to the process described above, in which a patient’s own blood cells are transformed into iPSCs and differentiated into healthy RPE cells on a specially designed patch.11 These stem cells must then be genetically modified via CRISPR technology to remove the pathogenic mutations.12,13 The patch is surgically implanted into the subretinal space, enabling potential integration into the visual circuitry and restoration of RPE function. The NYSCF expects that clinical trials will soon be under way.
For degenerative diseases of photoreceptors like RP, photoreceptor replacement has also proven to be possible. Takahashi et al successfully developed iPSC-derived retinal organoids that mimic the complex neuronal architecture of the retina.14 These 3D structures mature in vitro into stratified tissues that recapitulate key retinal architecture containing photoreceptors, bipolar cells, and other retinal neurons. Hirami et al transplanted allogeneic-derived retinal organoids into 1 eye of each of 2 patients with RP.15 The graft remained stable at 2 years after the operation, and progression of vision loss was slowed in the treated eye relative to the untreated eye in both patients. Once again, more research will be necessary to determine the efficacy of this approach in rescuing visual function.
Aside from cell replacement, stem cells may exert therapeutic effects through trophic factor secretion and immunomodulation. Park et al reported a phase 1 study of intravitreal injection of autologous CD34+ stem cells from bone marrow in patients with RP that resulted in stabilization or minor improvement in visual acuity at 6 months postinjection.16
Limitations of Stem Cell Therapy
Despite the eye’s immune privilege, immune rejection remains a persistent challenge in stem cell therapy, particularly with allogeneic grafts. Sugita et al suggests the use of human leukocyte antigen (HLA)–matched allogeneic iPSC-derived RPE cells to minimize the rejection risk.17 Their approach used cells from HLA-homozygous donors to reduce the need for long-term immunosuppression. On the other hand, Kashani et al showed that even HLA-mismatched RPE implants can survive without long-term immunosuppression, suggesting that immune tolerance to implants may be achievable under specific conditions.18
The delivery method also impacts the efficacy and safety of stem cell therapies. Two primary approaches have emerged: cell suspension injection, and the implantation of preformed cell sheets on scaffolds. The injection of cell suspensions is technically simpler but may result in uneven distribution and poor cell survival, whereas scaffold-based approaches can maintain cellular polarity and organization but require more complex surgical techniques.19 Humayun et al emphasized the importance of careful and accurate surgical technique in the placement of RPE scaffold implants to ensure proper positioning in the submacular space.6 Other safety considerations, particularly the risk of tumorigenesis with pluripotent stem cells, are also paramount.
There are also ethical considerations in stem cell use. Because ESC derivation involves the destruction of human embryos, for example, the use of ESCs in stem cell therapy has sparked ongoing moral and religious debates about the ethical use of these embryos.20 These debates have given rise to various regulatory frameworks across several countries, rendering collaborative international research efforts more complicated in fields not limited to ophthalmology.
Conclusion and Future Directions
Stem cell therapy harnesses the high regenerative potency of undifferentiated stem cells to replace diseased or dead cells. In recent years, stem cell therapy for retinal diseases has transitioned from preclinical investigation to early phase clinical trials with promising results. Both RPE replacement and photoreceptor transplantation strategies have shown the potential to preserve or restore visual function in conditions like AMD and RP, offering hope for conditions once considered irreversible. Future approaches may combine stem cell therapy with adjuvant treatment modalities, such as gene therapy or neuroprotective agents, to enhance efficacy in individualized, patient-specific treatment plans.
Advances in stem cell derivatives are becoming more commonplace in practice, optimized via gene editing for increased specificity and effectiveness.21 Gene correction in patient-derived iPSCs before differentiation and transplantation could one day address both the cellular degeneration and the underlying genetic defect.22 Additionally, recent research has begun to explore the possibility of directly reprogramming somatic cells into retinal cells without the need for these cells to pass through a pluripotent state. Woogeng et al induced the conversion of RPE-like cells directly from fibroblasts using a combination of transcription factors and small molecules.23 Direct reprogramming approaches could potentially mitigate some of the safety concerns associated with iPSCs.
Despite significant progress in the last decade, a few challenges remain in the clinical application of stem cell therapies for retinal diseases: namely, immune rejection and the safety of delivery mechanisms in human patients. Even when these challenges are overcome, further advances will be necessary to improve the effectiveness of cell replacement in restoring the vision of patients with AMD, RP, and other diseases. RP
References
1. Takahashi K, Yamanaka S. Induction of pluripotent stem cells from mouse embryonic and adult fibroblast cultures by defined factors. Cell. 2006;126(4):663-676. doi:10.1016/j.cell.2006.07.024
2. Carr AJ, Smart MJ, Ramsden CM, Powner MB, da Cruz L, Coffey PJ. Development of human embryonic stem cell therapies for age-related macular degeneration. Trends Neurosci. 2013;36(7):385-395. doi:10.1016/j.tins.2013.03.006
3. Maeda T, Mandai M, Sugita S, Kime C, Takahashi M. Strategies of pluripotent stem cell–based therapy for retinal degeneration: update and challenges. Trends Mol Med. 2022;28(5):388-404. doi:10.1016/j.molmed.2022.03.001
4. Singh MS, Park SS, Albini TA, et al. Retinal stem cell transplantation: Balancing safety and potential. Prog Retin Eye Res. 2020;75:100779. doi:10.1016/j.preteyeres.2019.100779
5. Strauss O. The retinal pigment epithelium in visual function. Physiol Rev. 2005;85(3):845-881. doi:10.1152/physrev.00021.2004
6. Humayun MS, Lee SY. Advanced retina implants. Ophthalmol Retina. 2022;6(10):899-905. doi:10.1016/j.oret.2022.04.009
7. Mandai M, Watanabe A, Kurimoto Y, et al. Autologous induced stem cell–derived retinal cells for macular degeneration. N Engl J Med. 2017;376(11):1038-1046. doi:10.1056/NEJMoa1608368
8. da Cruz L, Fynes K, Georgiadis O, et al. Phase 1 clinical study of an embryonic stem cell–derived retinal pigment epithelium patch in age-related macular degeneration. Nat Biotechnol. 2018;36(4):328-337. doi:10.1038/nbt.4114
9. Davis RJ, Alam NM, Zhao C, et al. The developmental stage of adult human stem cell–derived retinal pigment epithelium cells influences transplant efficacy for vision rescue. Stem Cell Reports. 2017;9(1):42-49. doi:10.1016/j.stemcr.2017.05.016
10. The New York Stem Cell Foundation. NYSCF advances toward a cell therapy for age-related macular degeneration. March 22, 2021. Accessed April 8, 2025. https://nyscf.org/resources/nyscf-advances-toward-a-cell-therapy-for-age-related-macular-degeneration/
11. Song MJ, Bharti K. Looking into the future: using induced pluripotent stem cells to build two and three dimensional ocular tissue for cell therapy and disease modeling. Brain Res. 2016;1638(Pt A):2-14. doi:10.1016/j.brainres.2015.12.011
12. Tsang SH, editor. CRISPR Genome Surgery in Stem Cells and Disease Tissues. Cambridge, MA: Academic Press, 2020.
13. Tsang, SH, editor. Stem Cell Biology and Regenerative Medicine in Ophthalmology. New York: Humana Press, 2013.
14. Takahashi M, Mandai M, Miyamoto N, et al. Organogenesis from stem cells in the eye. Cell Stem Cell. 2022;29(5):663-679.
15. Hirami Y, Mandai M, Sugita S, et al. Safety and stable survival of stem cell–derived retinal organoid for 2 years in patients with retinitis pigmentosa. Cell Stem Cell. 2023;30(12):1585-1596.e6. doi:10.1016/j.stem.2023.11.004
16. Park SS, Bauer G, Fury B, et al. Phase 1 study of intravitreal injection of autologous CD34+ stem cells from bone marrow in eyes with vision loss from retinitis pigmentosa. Ophthalmol Sci. 2024;5(1):100589. doi:10.1016/j.xops.2024.100589
17. Sugita S, Mandai M, Hirami Y, et al. HLA-matched allogeneic iPS cells–derived RPE transplantation for macular degeneration. J Clin Med. 2020;9(7):2217. doi:10.3390/jcm9072217
18. Kashani AH, Lebkowski JS, Hinton DR, et al. Survival of an HLA-mismatched, bioengineered RPE implant in dry age-related macular degeneration. Stem Cell Reports. 2022;17(3):448-458. doi:10.1016/j.stemcr.2022.01.001
19. Stanzel BV, Liu Z, Somboonthanakij S, et al. Human RPE stem cells grown into polarized RPE monolayers on a polyester matrix are maintained after grafting into rabbit subretinal space. Stem Cell Reports. 2014;2(1):64-77. doi:10.1016/j.stemcr.2013.11.005
20. Lo B, Parham L. Ethical issues in stem cell research. Endocr Rev. 2009;30(3):204-213. doi:10.1210/er.2008-0031
21. Kimbrel EA, Lanza R. Next-generation stem cells—ushering in a new era of cell-based therapies. Nat Rev Drug Discov. 2020;19(7):463-479. doi:10.1038/s41573-020-0064-x
22. Bassuk AG, Zheng A, Li Y, Tsang SH, Mahajan VB. Precision medicine: genetic repair of retinitis pigmentosa in patient-derived stem cells. Sci Rep. 2016;6:19969. doi:10.1038/srep19969
23. Woogeng IN, Kaczkowski B, Abugessaisa I, et al. Inducing human retinal pigment epithelium-like cells from somatic tissue. Stem Cell Reports. 2022;17(2):289-306. doi:10.1016/j.stemcr.2021.12.008